Copy link
Single Ventricle Physiology
Last updated: 02/09/2023
Key Points
- Single ventricle physiology (SVP) describes a circulation with complete mixing of pulmonary venous and systemic venous blood at the atrial or ventricular level. Oxygen saturation is the same in the aorta and the pulmonary artery.
- In SVP, ventricular output is the sum of pulmonary blood flow (Qp) and systemic blood flow (Qs). The distribution of systemic and pulmonary blood flow is dependent on the relative resistances to flow (both intra- and extracardiac) into the two parallel circuits.
- SVP can exist in patients with either one well-developed ventricle and one hypoplastic ventricle or two well-formed ventricles.
Introduction
SVP is a very specific and precise term used to describe a circulation wherein complete mixing of pulmonary venous and systemic venous blood occurs at the atrial or ventricular level and where the ventricle(s) then distribute output to both the systemic and pulmonary vascular beds.
As a result of this physiology, the:
- Ventricular output is the sum of pulmonary blood flow (Qp) and systemic blood flow (Qs).
- Distribution of systemic and pulmonary blood flow is dependent on the relative resistances to flow (both intra- and extracardiac) into the two parallel circuits.
- Oxygen saturations are the same in the aorta and the pulmonary artery.
Anatomy
- SVP can exist in patients with one well-developed ventricle and one hypoplastic ventricle as well as in patients with two well-formed ventricles (Table 1).
- The term SVP should NOT be used to characterize all patients with single ventricle anatomy as a sizable portion of these patients have either bidirectional Glenn or Fontan physiology.
- Patients with two well-formed ventricles may initially or ultimately be candidates for a biventricular repair.
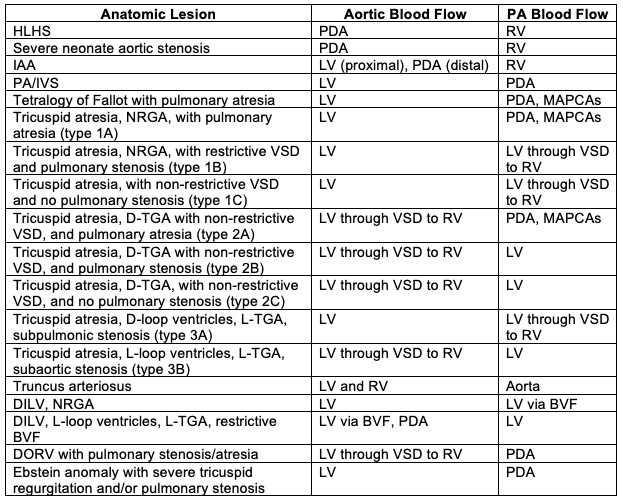
Table 1. Anatomic lesions which manifest SVP. Abbreviations: BVF, bulboventricular foramen; D, dextro; DILV, double inlet left ventricle; HLHS, hypoplastic left heart syndrome; IAA, interrupted aortic arch; L, levo; LV left ventricle; MAPCAs, multiple aortopulmonary collateral arteries; NRGA, normally related great arteries; PA, pulmonary artery, PA/IVS, pulmonary atresia with intact ventricular septum; PDA, patent ductus arteriosus; RV, right ventricle; TGA, transposition of the great arteries; VSD, ventricular septal defect; DORV, double outlet right ventricle. Reproduced with permission from Nasr, VG, DiNardo JA. Pediatric cardiac anesthesia handbook. New Jersey: Wiley; 2017.
- As an example of SVP, the anatomy, chamber oxygen saturations, and blood flows in a patient with tricuspid atresia are summarized below in Figure 1.
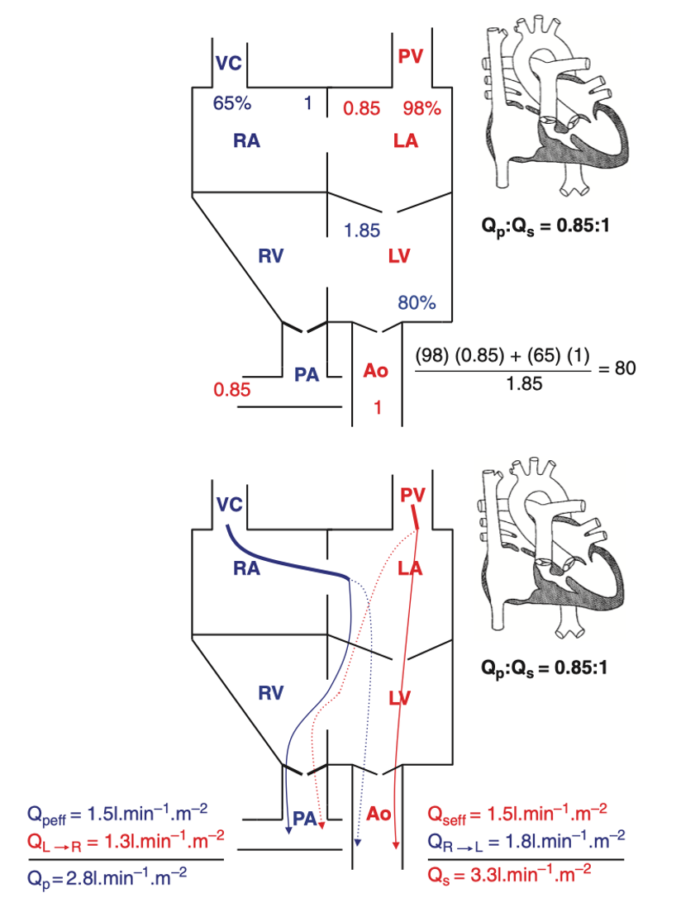
Figure 1. Blood flows and saturations in tricuspid atresia 1B. Effective pulmonary and systemic blood flows are equal and shown by solid lines. Shunted pulmonary and systemic blood flows are shown as dotted lines. It should be emphasized that effective pulmonary and effective systemic blood flows are always equal in any cardiac lesion, regardless of complexity. Reproduced with permission from Nasr, VG, DiNardo JA. Pediatric cardiac anesthesia handbook. New Jersey: Wiley; 2017.
Physiology
- With SVP, the arterial saturation (SaO2) will be determined by the relative volumes and saturations of pulmonary venous and systemic venous blood flows that have mixed and reached the aorta. This is summarized in the following equation:

This is illustrated in the Figure 1 where: SaO2= [(65)(1) + (98)(0.85)]/(1.85) = 80%
- In SVP, three variables will determine arterial saturation:
- The ratio of total pulmonary to total systemic blood flow (Qp:Qs): When Qp:Qs is high, a greater proportion of the mixed blood will consist of saturated blood (pulmonary venous blood) than of desaturated blood (systemic venous blood). Figure 2b demonstrates the increase in arterial saturation that occurs with increases in pulmonary blood flow relative to systemic blood flow.
- Systemic venous saturation: For a given QP:QS and pulmonary venous saturation, a decrease in systemic venous saturation will result in a decreased arterial saturation. Decreases in systemic venous saturation occur as the result of decreases in systemic oxygen delivery or increases in systemic oxygen consumption. Systemic oxygen delivery is the product of systemic blood flow and arterial oxygen content. Arterial oxygen content is dependent on the hemoglobin concentration and the arterial saturation.
- Pulmonary venous saturation: In the absence of large intrapulmonary shunts and/or V/Q mismatch, pulmonary venous saturation should be close to 100% on room air. In the presence of pulmonary parenchymal disease, pulmonary venous saturation may be reduced. The V/Q mismatch component of pulmonary venous desaturation will be largely eliminated with a FiO2 of 1.0, while the intrapulmonary shunt contribution will not be eliminated. For any given systemic venous saturation and QP:QS, a reduction in pulmonary venous saturation will result in a decreased arterial saturation.
- Systemic O2 delivery (CaO2 • Qp) is a complex function of cardiac output (CO), pulmonary venous O2 content (CpvO2), systemic O2 consumption (CVO2), and Qp:Qs described by the equation:
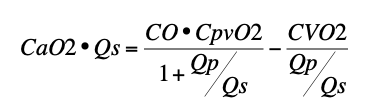
- Neither SaO2 nor the equation 25/(95-SaO2) reliably predicts Qp:Qs.
- These relationships are summarized in Figure 2.
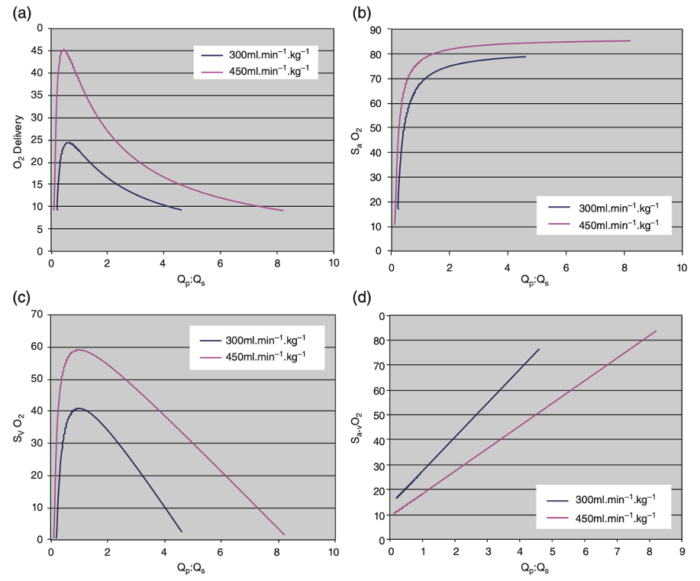
Figure 2. (a) Systemic oxygen (O2) delivery (mL/kg/min versus Qp:Qs for two different systemic ventricular outputs (300 mL/kg/min and 450 mL/kg/min) in SVP. Oxygen consumption is assumed to be 9 mL/kg/min and SpVO2= 95 %. It is clear that systemic oxygen delivery peaks just below a Qp:Qs of 1:1 and declines rapidly above or below this narrow peak. (b) SaO2 versus Qp:Qs for two different systemic ventricular outputs (300 mL/kg/min and 450 mL/kg/min in SVP. Oxygen consumption is assumed to be 9 mL/kg/min and SpVO2= 95%. It is clear that SaO2 remains at or near 80% over a wide range of Qp:Qs and consequently is a very poor surrogate measure of Qp:Qs. (c) SvO2 versus Qp:Qs for two different systemic ventricular outputs (300 mL/kg/min and 450 mL/kg/min) in SVP. Oxygen consumption is assumed to be 9 mL/kg/min and SpVO2= 95%. SvO2 decreases precipitously outside a narrow range of Qp:Qs near 1.0. (d) Sa-vO2 versus Qp:Qs for two different systemic ventricular outputs (300 mL/kg/min and 450 mL/kg/min) in SVP. Oxygen consumption is assumed to be 9 mL/kg/min and SpVO2= 95%. SvO2 increases linearly as Qp:Qs increases. The slope of this relationship decreases as systemic ventricular output increases. Reproduced with permission from Nasr VG, DiNardo JA. Pediatric cardiac anesthesia handbook. New Jersey: Wiley; 2017.
Medical and Surgical Management
Medical Management
A continuous infusion of prostaglandin E1 (PGE1) is used to maintain ductal patency in patients with ductal-dependent blood flow (either systemic or pulmonary). PGE1 can be discontinued in any patient determined by an echocardiogram to have a reliable nonductal source of systemic or pulmonary blood flow. Ductal patency generally produces unrestrictive pulmonary blood flow.
Surgical Management
The initial palliative procedures described here are applicable to any patient with SVP.
Initial Procedures to Optimize Pulmonary Blood Flow
- Patients with ductal-dependent pulmonary blood flow will require the creation of a consistent source of pulmonary blood flow, either via stenting of the patent ductus arteriosus or the surgical placement of an aortopulmonary shunt (i.e., modified Blalock-Thomas-Taussig shunt), or in the case of HLHS, an RV-PA conduit (Sano).
- Patients with unrestricted pulmonary blood flow are at risk for compromise of systemic oxygen delivery due to high pulmonary blood flow in the setting of fixed cardiac output and, ultimately, for the development of pulmonary arterial hypertension. These patients need restriction of pulmonary blood flow:
- In patients with high pulmonary blood flow and significant intracardiac sources of pulmonary blood flow, PDA closure may appropriately limit pulmonary blood flow.
- More commonly, it will be necessary to mechanically restrict pulmonary blood flow and reduce QP:QS and the volume load on the systemic ventricle by banding the pulmonary artery. PA banding is contraindicated in patients where aortic blood flow is derived from a restrictive intracardiac pathway from the systemic ventricle or where this pathway is likely to become restrictive once the ventricular volume load is reduced by a PA band. These patients require a Damus-Kaye-Stansel (DKS) procedure (see below) to provide an unobstructed pathway to the aorta from the systemic ventricle.
Initial Procedures to Optimize Pulmonary Venous Blood Flow
- In instances in which pulmonary venous blood must cross the atrial septum to reach the systemic ventricle, the atrial septum must be nonrestrictive. Creating a nonrestrictive atrial septum may occur in the cardiac catheterization laboratory using a Rashkind-Miller balloon atrial septostomy +/- stenting. A surgical atrial septectomy to create a nonrestrictive atrial septum (common atrium) can also be performed at the time of initial palliation in procedures that require CPB.
Initial Procedures to Optimize Systemic Blood Flow
- When the pathway to the aorta from the systemic ventricle is obstructed or when the LV and proximal/transverse aorta are hypoplastic (as with HLHS), a Damus-Kaye-Stansel (DKS) procedure alone or in conjunction with an aortic arch reconstruction (as with HLHS), can be used. The pulmonary artery is transected just proximal to its bifurcation, and the proximal end of the pulmonary artery is reanastomosed end to side or side to side to the ascending aorta. This provides systemic ventricle to aortic continuity. Pulmonary blood flow is then supplied to the main PA as described above.
Anesthesia Considerations
Overall Goals
- Patients with SVP should be cared for in tertiary cardiac centers with appropriate anesthesia, critical care, catheterization, and surgical capabilities.
- The primary goal in the management of patients with SVP is the prevention of end-organ injury. Systemic oxygen delivery and perfusion pressures are optimized by balancing the systemic and pulmonary circulations (QP:QS ~1:1). QP:QS > 1:1 is associated with a decrease in systemic oxygen delivery due to a decrease in systemic blood flow. QP:QS < 1:1 is associated with a decrease in systemic oxygen delivery due to a decrease in systemic oxygen content.
Ventilation
- Excessive Qp should be avoided by titrating ventilation to maintain mild hypercarbia (PaCO2 45-55mmHg), a PaO2 of 40-45mmHg, and a SaO2 of 75-80%.
- High FiO2 should be avoided.
- FRC should be maintained (tidal volume 8-12 mL/kg, 2-5cm H20 of PEEP) to avoid atelectasis and intrapulmonary shunting.
Hemodynamic Support
- For ductal-dependent lesions, a prostaglandin infusion should be continued until a definitive source of pulmonary (or systemic) blood flow is established.
- Inotropic support with dopamine or epinephrine may be needed to support cardiac output and maintain an adequate diastolic pressure in the setting of an unrestricted right-to-left shunt.
- Systemic vasodilator therapy, such as milrinone, decreases SVR and may help to promote Qs and improve DO2.
- DO2 is highly dependent on hemoglobin concentration. A hematocrit of ~40% should be targeted.
References
- Nasr, VG, DiNardo JA. Pediatric cardiac anesthesia handbook. New Jersey: Wiley; 2017.
- Francis DP, Willson K, Thorne SA, et al. Oxygenation in patients with a functionally univentricular circulation and complete mixing of blood: are saturation and flow interchangeable? Circulation. 1999;100(21):2198-203. PubMed
- Barnea O, Santamore WP, Rossi A, et al. Estimation of oxygen delivery in newborns with a univentricular circulation. Circulation. 1998;98(14):1407-13. PubMed
- Li J, Zhang G, McCrindle BW, et al. Profiles of hemodynamics and oxygen transport derived by using continuous measured oxygen consumption after the Norwood procedure. J Thorac Cardiovasc Surg. 2007;133(2):441-8. PubMed
- Photiadis J, Sinzobahamvya N, Fink C, et al. Optimal pulmonary to systemic blood flow ratio for best hemodynamic status and outcome early after Norwood operation. Eur J Cardiothorac Surg. 2006;29(4):551-6. PubMed
Other References
- Ross FJ. Introduction to congenital heart disease. Part 3. OpenAnesthesia. Published September 1, 2018. Accessed February 9, 2023. Link
Copyright Information
This work is licensed under a Creative Commons Attribution-NonCommercial-NoDerivatives 4.0 International License.