Copy link
Inhaled Anesthetic Agents: Pharmacodynamics
Last updated: 03/28/2023
Key Points
- Inhaled anesthetic agents cause dose-dependent decreases in mean arterial pressure primarily by decreasing systemic vascular resistance.
- Inhaled anesthetics depress ventilation and can blunt the ventilatory response to hypoxia and hypercapnia.
- Increases in cerebral blood flow and intracranial pressure, with reductions in cerebral metabolic rate, can occur with inhaled anesthetics at 1.0 minimum alveolar concentration (MAC).
- Inhaled anesthetic agents produce muscular relaxation and attenuate neuromuscular blockade. They can also trigger malignant hyperthermia in susceptible patients.
Effects on Organ Systems
Cardiovascular
- Mean arterial pressure (MAP)
- There is a dose-dependent decrease with all volatile agents due to a decrease in systemic vascular resistance (SVR), and a minimal decrease in cardiac output (CO).1
- Halothane decreases MAP entirely by depressed cardiac output.
- Nitrous oxide has minimal effect on MAPs due to sympathetic stimulation.
- Heart rate (HR)
- Heart rate increases with isoflurane (>0.25 MAC), desflurane (>1.0 MAC), and less effect seen with sevoflurane due to attenuation of the baroreceptor reflex (>1.5 MAC).2
- Rapid increases in inspired concentrations of desflurane and isoflurane can lead to transiently increased HR, MAP, and catecholamine levels.
- Cardiac index (CI)
- Cardiac index is minimally affected by inhaled anesthetics and mild increase in ejection fraction with desflurane.
- Nitrous oxide is a direct myocardial depressant in vitro. However, in vivo, cardiac output, MAP, and HR are unchanged or mildly increased due to sympathetic stimulation.
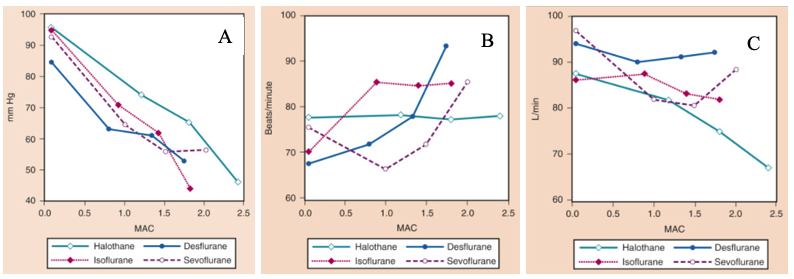
Figure 1. The effects of increasing concentrations (MAC) of halothane, isoflurane, desflurane, and sevoflurane on mean arterial pressure (mm Hg) (A), heart rate (beats/minute) (B), and cardiac index (L/min) (C), when administered to healthy volunteers. MAC, Minimum alveolar concentration. From Cahalan MK. Hemodynamic effects of inhaled anesthetics. Review Courses. Cleveland: International Anesthesia Research Society; 1996:14-18
- Other
- Pulmonary vascular resistance (PVR) is increased with nitrous oxide.
- QT interval: inhaled anesthetics can prolong QT interval and should be used carefully in susceptible patients (long QT syndrome).3
- Inhaled anesthetic agents decrease myocardial oxygen consumption and coronary vasodilation (seen more with isoflurane) to improve myocardial oxygenation.
- Inhaled anesthetics have been shown to exert a protective effect on myocardial tissue against ischemic injury.4
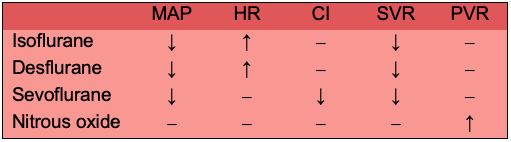
Table 1. Cardiac effects of inhaled anesthetics. Abbreviations: MAP, mean arterial pressure; HR, heart rate; CI, cardiac index; SVR, systemic vascular resistance; PVR, pulmonary vascular resistance.
Respiratory
- Minute ventilation
- ↑ respiratory rate (RR), ↓ tidal volume (TV) → overall decrease in alveolar ventilation leading to increasing PaCO2 levels.
- Airway reflexes: the pungency of desflurane and isoflurane can irritate the airways and precipitate coughing, laryngospasm, or bronchospasm on induction.
- Bronchial effects: inhaled anesthetics can produce mild bronchodilation at higher concentrations due to beta-2 receptor stimulation.5 This effect is most pronounced with sevoflurane.
- All inhaled anesthetics blunt the ventilatory response to hypoxia and hypercapnia.
- Inhaled anesthetics may attenuate hypoxic pulmonary vasoconstriction.6
- Inhaled anesthetics may depress mucociliary function in the airway.
Cerebral
- Cerebral blood flow (CBF): increased with inhaled anesthetics at 1.0 MAC.
- Intracranial pressure (ICP): increased with inhaled anesthetics above 1.0 MAC.
- Cerebral metabolic rate of oxygen (CMRO2): decreased with isoflurane, sevoflurane, and desflurane. CMRO2 is mildly increased with nitrous oxide.
- Inhaled anesthetics preserve cerebral vasculature response to changes in PaCO2.
- Electroencephalogram (EEG): inhaled anesthetics can produce burst suppression or an isoelectric EEG pattern at more than 2 MAC.
- Evoked potentials: ↓ in amplitude, ↑ latency of all cortical somatosensory evoked potentials with inhaled anesthetics. Motor evoked potentials are significantly depressed.
- Analgesia: some effect with nitrous oxide.
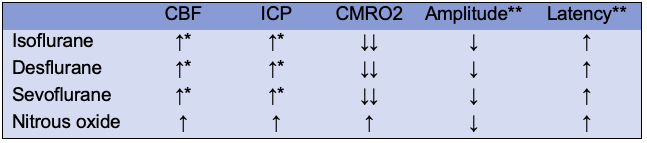
Table 2. Central nervous system effects of inhaled anesthetics. Abbreviations: CBF, cerebral blood flow; ICP, intracranial pressure; CMRO2, cerebral metabolic rate of oxygen. *Unchanged until concentration of 1 MAC (Minimal alveolar concentration), **Electroencephalogram at 1.0 MAC.
Neuromuscular
- Inhaled anesthetics produce dose-dependent relaxation of skeletal and smooth muscle by inhibiting nicotinic acetylcholine receptors.
- All inhaled anesthetics potentiate and reduce the required dose of neuromuscular blockers. Nitrous oxide has minimal effect on neuromuscular blockade.
- Desflurane > sevoflurane > isoflurane
- Uterine smooth muscle
- All inhaled anesthetics are potent dose-dependent uterine relaxants.
- Nitrous oxide has minimal effect on uterine musculature.
- Malignant hyperthermia: triggered by all inhaled anesthetics except nitrous oxide.
Renal
- Inhaled anesthetics can decrease renal blood flow, glomerular filtration rate, and urinary output.
Hepatic
- Inhaled anesthetics cause a dose-dependent decrease in portal vein flow proportional to cardiac output, while hepatic blood flow and total oxygen delivery are maintained.
- Nitrous oxide: possible decrease in hepatic blood flow, less than inhaled anesthetics.
- Although inhaled anesthetics can cause transient changes in liver function tests, persistent changes and severe immune-mediated liver injury is very rare.
Gastrointestinal
- All inhaled anesthetics increase the risk of postoperative nausea and vomiting.
- Gastrointestinal smooth muscle contraction can contribute to nausea, emesis, and ileus.
Ocular
- Inhaled anesthetics may decrease intraocular pressure (IOP).
- Nitrous oxide may increase IOP.
References
- McKay RE. Inhaled Anesthetics. In: Pardo MC, Miller RD. Basics of Anesthesia. Philadelphia, PA 7th ed. Elsevier. 2018: 83-103.
- Malan Jr. PT, DiNardo JA, Isner JR, et al. Cardiovascular effects of sevoflurane compared with those of isoflurane in volunteers. Anesthesiology. 1995;83(5):918-28. PubMed
- Booker PD, Whyte SD, Ladusans EJ. Long QT syndrome and anaesthesia. Br J Anaesth. 2003;90(3):349-366. PubMed
- De Hert SG, Cromheecke S, ten Broecke PW, et al. Effects of propofol, desflurane, and sevoflurane on recovery of myocardial function after coronary surgery in elderly high-risk patients. Anesthesiology. 2003;99(2):314-23. PubMed
- Rooke GA, Choi J-H, Bishop MJ. The effect of isoflurane, halothane, sevoflurane, and thiopental/nitrous oxide on respiratory system resistance after tracheal intubation. Anesthesiology. 1997;86(6):1294-99. PubMed
- 6. Lumb AB, Slinger P. Hypoxic pulmonary vasoconstriction: physiology and anesthetic implications. Anesthesiology. 2015;122(4):932-46. PubMed
Copyright Information
This work is licensed under a Creative Commons Attribution-NonCommercial-NoDerivatives 4.0 International License.