Copy link
Inhaled Anesthetics in Clinical Use
Last updated: 04/26/2023
Key Points
- Variable-bypass vaporizers are used to deliver most inhaled anesthetics and depend on relative gas flow and the anesthetic agent’s vapor pressure.
- Desflurane has a high vapor pressure and boiling point near room temperature, requiring a specialized vaporizer that heats and injects the agent for predictable output.
- Isoflurane, sevoflurane, and desflurane are the most commonly used agents today. Nitrous oxide is a useful anesthesia adjuvant but has a unique side-effect profile.
- Inhaled anesthetics are potent greenhouse gases, and efforts should be made to minimize their environmental impact.
Vapor Pressure
Saturated Vapor Pressure (SVP)
- SVP is the pressure exerted by molecules from a volatile substance that escape the liquid phase and become vapor (when the liquid and vapor are at equilibrium) (Figure 1).
- This pressure is unique to each agent and is directly proportional to the number of molecules of gas within a container at a certain temperature.
- Effect of temperature: At a higher temperature, the SVP will increase due to higher kinetic energy and greater conversion to a gas phase.
- SVP is not affected by changes in atmospheric pressure.
- Sevoflurane, isoflurane, desflurane, and halothane are liquid, while nitrous oxide is a gas at ambient pressures.
Boiling point is the temperature at which vapor pressure equals ambient pressure, and the liquid substance will rapidly vaporize.
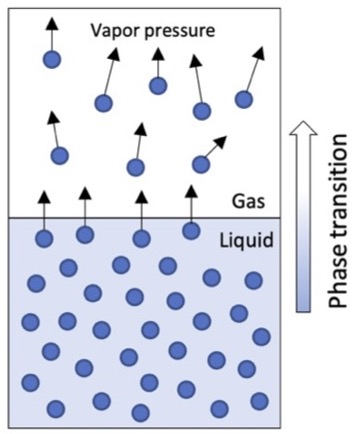
Figure 1. Vapor pressure exerted by molecules that transition from liquid to gas.
Anesthetic Vaporizers
Variable-Bypass Vaporizer (VBV)
- Mechanism: Two streams of gas are used, with one contacting a reservoir (sump) with a volatile anesthetic and another bypassing the reservoir. The reservoir stream will pick up anesthetic vapor particles and exit to be diluted by the bypass channel (Figure 2A).
- The concentration of the anesthetic in the gas exiting the vaporizer is determined by the relative gas flow into the reservoir channel (splitting ratio) and the vapor pressure of the specific anesthetic agent.
- Variable-bypass vaporizers compensate for changes in temperature to maintain constant output.
Heated Vaporizer
- Desflurane has a high vapor pressure and boiling point near room temperature, making the output concentration delivered by a VBV unpredictable.
- The Tec 6 vaporizer (Datex-Ohmeda) heats desflurane to 39°C (2 atms of pressure) for a constant, predictable output concentration (Figure 2B).
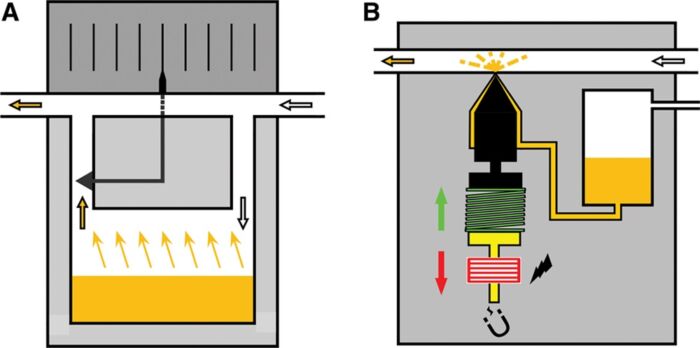
Figure 2A. General principle of variable bypass vaporizer. When the wheel (dial) is turned, more or less fresh gas is directed through the vaporizing chamber containing the liquid agent (orange). Gas exiting the vaporizing chamber is saturated with agent (at the prevailing temperature) and mixes with gas bypassing the vaporizing chamber. B, Injector principle. The liquid agent (orange) is pressurized, which makes the system suitable for desflurane. The liquid agent is injected into a heated chamber (where it evaporates and mixes with fresh gas) or directly into the breathing system via a nozzle. In the resting position, a spring (green) pushes the ferromagnetic plunger (yellow) into the nozzle. When the microprocessor directs current through the coiled wire surrounding the ferromagnetic plunger (yellow), the plunger is moved backwards (red arrow), and the liquid agent is injected. Used with permission from Hendrickx J, De Wolf A. The anesthesia workstation: Quo vadis? Anesth Analg. 2018;127(3):671-5.
Calculating the Amount of Anesthetic Delivered
- The volume of anesthetic can be calculated within a volume of fresh gas flow:
(VA = agent vapor volume, SVP = saturated vapor pressure, PT = total pressure [usually atmospheric], VC = carrier gas volume)
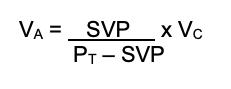
- Once the volume is known, the total % concentration can be calculated:

Effect of Altitude on Vaporizer Output
- Variable-bypass vaporizer: Maintains constant partial pressure of inhaled anesthetic. As the altitude increases and barometric pressure decreases, the volume percent concentration will increase, but the partial pressure will be unchanged.
- The anesthetic effect is determined by partial pressure in the brain.
- No adjustments in dosages are required.
- Heated vaporizer: maintains constant volume concentration output. As ambient pressure decreases and output volume remains unchanged, the partial pressure of the anesthetic will drop.
- Results in an underdosing of the anesthetic. Therefore, the output will need to be increased.
- Calibration formula1:
Required dial setting (%) = desired % x (760 mmHg/ local barometric pressure [mmHg])
Inhaled Anesthetics in Clinical Use
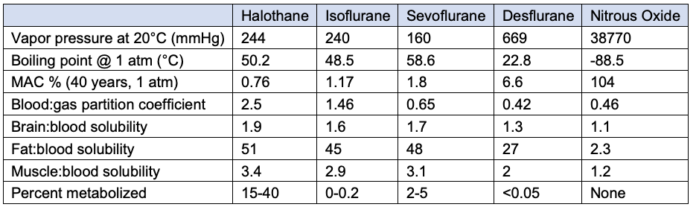
Table 1. Comparison of inhaled anesthetics. MAC: minimum alveolar concentration. Solubility coefficient of 0.65 means that at steady state, 1ml of blood will have 0.65 as much of sevoflurane compared to 1.0 in the alveolar gas. Adapted from Forman SA, Ishizawa Y. Inhaled Anesthetic Uptake, Distribution, Metabolism, and Toxicity. In: Miller RD, Gropper MA, et al. Miller's Anesthesia. Philadelphia, PA 9th ed. Elsevier. 2019: 509-39.
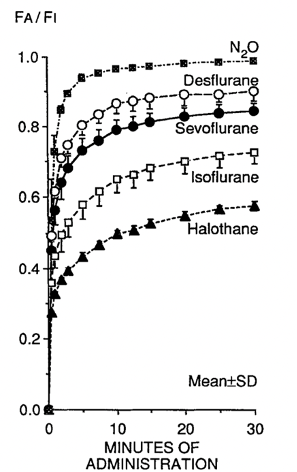
Figure 3. Comparison of pharmacokinetics of inhaled anesthetics. The blood-gas partition coefficient is the principal determinant of the rate increasing alveolar concentration to inspired concentration FA/FI which determines the speed of induction. Used with permission from Yasuda N, et al. Comparison of kinetics of sevoflurane and isoflurane in humans. Anesth Analg. 1991; 72:316-24.
Halothane
- The first modern halogenated inhaled anesthetic (introduced in 1955)
- The most potent of modern inhaled anesthetics
- Not pungent, useful for inhalational induction
- Most potent bronchodilator of all anesthetics
- Not widely used in the United States due to adverse effects
- Cardiac dysrhythmias and direct myocardial depression
- Halothane hepatitis: fulminate liver failure, likely resulting from immune-mediated hepatoxicity (rate of 1:20,000)2
Isoflurane
- Highly soluble, more potent than sevoflurane and desflurane
- Highly pungent odor
- Inexpensive
- Historic concern for “coronary steal” phenomenon due to coronary vasodilation, however, not clinically valid
Sevoflurane
- Intermediate solubility, faster induction, and elimination than halothane or isoflurane
- Non-pungent odor, useful for inhalational induction
- Historical concern for degradation by carbon dioxide absorbents into a vinyl ether called Compound A, resulting in nephrotoxicity; however, this is no longer a clinical concern in humans.
Desflurane
- Lowest blood: gas solubility, resulting in rapid induction and elimination
- Least potent, requiring higher concentrations for clinical effect
- Highly pungent odor causes airway irritation (laryngospasm, bronchospasm, breath-holding)
- Rapid increases in concentration can lead to transient increases in heart rate.
- Greater formation of carbon monoxide by desiccated carbon dioxide absorbents
- More expensive than other inhaled anesthetics
- Significantly worse environmental impact (see Table 2)
Nitrous Oxide (N2O)
- Rapid onset and offset due to very low solubility
- Low potency and unable to achieve 1.0 MAC, commonly used as an adjuvant
- NMDA antagonism may produce analgesic effects.
- N2O is 30 times more soluble than nitrogen, diffusing into air-filled cavities.
- Contraindicated in patients with pneumothorax, air embolism, intestinal obstruction, middle ear surgery, pneumocephalus
- Can increase pressure on equipment cuffs (i.e., endotracheal tube, supraglottic airway, pulmonary artery catheter), causing damage to surrounding tissue
- Inhibits DNA synthesis by inactivating vitamin-B12-dependent enzymes, which are critical cofactors in the activity of methionine synthase
- Diffusion hypoxia: rapid diffusion of N2O from blood to alveoli dilutes the concentration of alveolar oxygen and can result in hypoxia.
- Clinical effects can be avoided by administration of supplemental oxygen for the first 5-10 minutes of recovery.2
- Concentration effect: The rapid uptake of N2O results in a smaller relative decrease in concentration because of an accompanying decrease in alveolar volume.
- Second gas effect: when administering an anesthetic gas mixture with high concentrations of N2O, rapid uptake of N2O will reduce the alveolar volume and increase the concentration of the other alveolar gases and augment their uptake.
Environmental Impact of Inhaled Anesthetics
- Inhaled anesthetics are significant contributors to greenhouse gas emissions. This has resulted in calls to mitigate their environmental impact by reducing fresh gas flow during use, limiting or eliminating desflurane from practice, and advancing gas recapture/reuse technology.3
- The different inhaled anesthetics have disproportionate climate impacts, with significantly greater environmental harm from desflurane (Table 2).
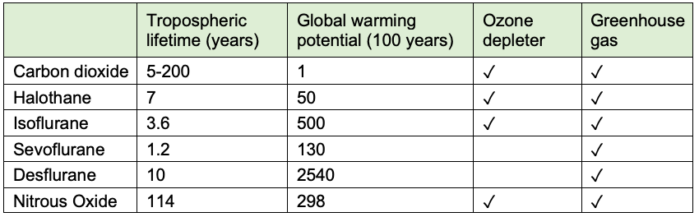
Table 2. Environmental effects of inhaled anesthetics. Used with permission from Varughese, et al. Environmental and occupational considerations of anesthesia: A narrative review and update. Anesth Analg. 2021;133(4):826-35.
References
- Brockwell R.C., Andrews J.J.: Vaporizers (in delivery systems for inhaled anesthetics). In: Barash P.G. Cullen B.F. Stoelting R.K. Cahallan M. Clinical Anesthesia. Philadelphia PA 8th ed. Lippincott Williams & Wilkins. 2009: 667-669.
- Forman SA, Ishizawa Y. Inhaled Anesthetic Uptake, Distribution, Metabolism, and Toxicity. In: Gropper M, Cohen NH, Miller RD, et al. Miller's Anesthesia. Philadelphia, PA 9th ed. Elsevier. 2019: 509-539.
- Devlin‐Hegedus JA, McGain F, Harris RD, Sherman JD. Action guidance for addressing pollution from inhalational anaesthetics. Anaesthesia. 2022;77(9):1023-1029.
- Varughese S, Ahmed R. Environmental and occupational considerations of anesthesia: A narrative review and update. Anesth Analg. 2021;133(4):826-35. PubMed
- Hendrickx JFA, De Wolf AM. The anesthesia workstation: Quo vadis? Anesth Analg. 2018;127(3):671-675. PubMed
Copyright Information
This work is licensed under a Creative Commons Attribution-NonCommercial-NoDerivatives 4.0 International License.