Copy link
Anesthesia for Laparoscopic and Robotic Surgery
Last updated: 07/16/2024
Key Points
- Minimally invasive surgery (including laparoscopic and robotic-assisted surgery) is increasingly common and has become the standard of care for some surgical procedures, given its multiple beneficial postoperative outcomes.
- Abdominal insufflation, which is necessary for laparoscopic operations, causes many physiologic changes, often related to hypercarbia—most notably to the circulatory and pulmonary systems—requiring specific management by the anesthesia provider.
- Both hemodynamic and pulmonary complications can occur during laparoscopic surgeries.
Overview of Laparoscopic and Robotic Surgery1-5
- A brief history of laparoscopic and robotic surgery:
- The first laparoscopic surgery was performed on a human in 1910.
- The first laparoscopic appendectomy was performed in 1981.
- In 1987, the first laparoscopic procedure utilizing a robotic system was used to perform a cholecystectomy.
- The Da Vinci surgery system was approved by the United States Food and Drug Administration for general laparoscopic surgery in 2000.
- Minimally invasive surgery (MIS) has several advantages:
- MIS decreases the perioperative stress response.
- Less tissue manipulation and smaller incisions result in fewer adhesions, faster recovery, and better postoperative pain control.
- This also leads to fewer wound complications, including hernias and infections.
- There is less bleeding, which results in a decreased need for blood transfusion.
- Patients have an overall faster recovery and shorter hospital stays.
- Use of MIS:
- MIS is currently the standard of care for many intraabdominal procedures, including cholecystectomy and appendectomy.
- There are an estimated 13-15 million laparoscopic procedures worldwide each year.
- There have been more than 10 million robotic procedures performed with the Da Vinci surgical system worldwide.
Physiologic Effects
Cardiovascular Effects1,2,6,7,8,9
- Hypercarbia from insufflation leads to a neuroendocrine response that results in increased catecholamine, vasopressin, and cortisol secretion. This leads to a relative increase in mean arterial pressure, systemic vascular resistance, and central venous pressure. Direct compression of the aorta by intraabdominal insufflation also leads to an increase in systemic vascular resistance.
- Increased intrathoracic pressure due to pneumoperitoneum leads to an increase in cardiac filling pressures.
- There can be a variation in cardiac filling volumes due to the following:
- Compression of the liver and spleen can lead to increased intravascular volume.
- The pneumoperitoneum can also potentially reduce preload and venous return through compression of the inferior vena cava.
- Upon the initiation of pneumoperitoneum, the cardiac index may decrease. However, this normalizes after approximately 15 minutes.
- The peritoneal stretch from insufflation can lead to a vagal response and bradyarrhythmias. Hypercarbia can also cause tachyarrhythmias.
- Acidosis from hypercarbia can result in decreased cardiac contractility, sensitization to arrhythmias, and systemic vasodilation.
- Positioning effects
- Patients are often placed in reverse Trendelenburg for upper gastrointestinal surgeries, including bariatric surgeries and cholecystectomies.
- This leads to venous pooling, decreased venous return to the heart, and thus hypotension, which is more pronounced in hypovolemic patients.
- Trendelenburg positioning is often used for surgeries in the pelvis and can result in increased venous return and cardiac filling pressures.
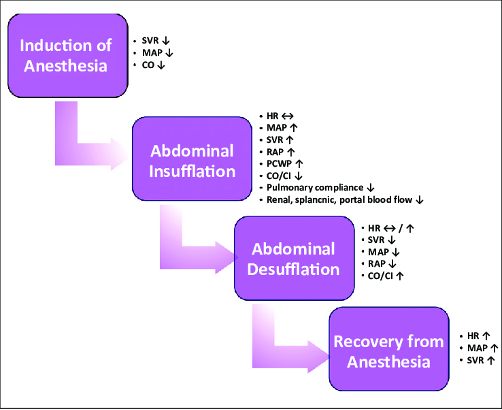
Figure 1. Hemodynamic effects of laparoscopic surgery. Abbreviations: SVR = systemic vascular resistance, MAP = mean arterial pressure, CO = cardiac output, HR = heart rate, RAP = right atrial pressure, PCWP = pulmonary capillary wedge pressure, CI = cardiac index. Gokdemir BN, Cekmen N. Laparoscopic surgery and anesthesia. JARSS. 2023;31(3):173-87.3 CC BY NC 4.0. Link
Pulmonary Effects1,2,3,6,7
- Cephalad displacement of the mediastinum and diaphragm leads to decreased functional residual capacity and pulmonary compliance.
- This results in shunting and V/Q mismatch.
- Increased atelectasis results in increased peak airway pressures.
- This is made worse by Trendelenburg positioning.
- Minute ventilation must be increased to compensate for the absorption of carbon dioxide (CO2).
- Pulmonary vasoconstriction results from CO2 absorption and physical compression of the lungs.
- In obese patients, there is an increased alveolar-arterial oxygen gradient (arterial oxygen decreases) when in the Trendelenburg position. Insufflation may slightly reverse this effect.10
- CO2 absorption plateaus at ~1 hour.
- Subcutaneous emphysema can result from insufflation.
- Insufflation can directly result in endobronchial migration of the endotracheal tube (ETT) secondary to cephalad movement of carina or increased ETT cuff pressure.
- Elevated CO2 levels shift the oxyhemoglobin dissociation curve to the right via the Haldane effect. This helps to deliver oxygen to the tissues and results in slightly less ischemia than would be expected.
Neuroendocrine Effects7
- Catecholamine release leads to activation of the renin-angiotensin-aldosterone system. This leads to antidiuretic hormone release.
- Peritoneal stretch leads to vagal stimulation.
Regional Circulatory Changes1,3,7
- Splanchnic circulation is decreased by mechanical and neuroendocrine effects.
- This results in reduced total hepatic blood flow and bowel perfusion; however, it is counteracted by splanchnic vasodilation caused by hypercarbia. Thus, the overall change to splanchnic circulation is not clinically significant.
- Parenchymal compression leads to a decrease in renal perfusion and, thus, urine output.
- Attempts should be made to maintain insufflation pressures of less than 15 mmHg to allow for the normalization of renal function and urine output.
- Hypercarbia leads to increased cerebral blood flow and intracranial pressures.
- This is likely clinically insignificant; however, strict normocapnia should be maintained in patients with cerebrovascular disorders or intracranial mass lesions.
- Trendelenburg positioning leads to increased intraocular pressure, which can last ~1 hour after surgery.
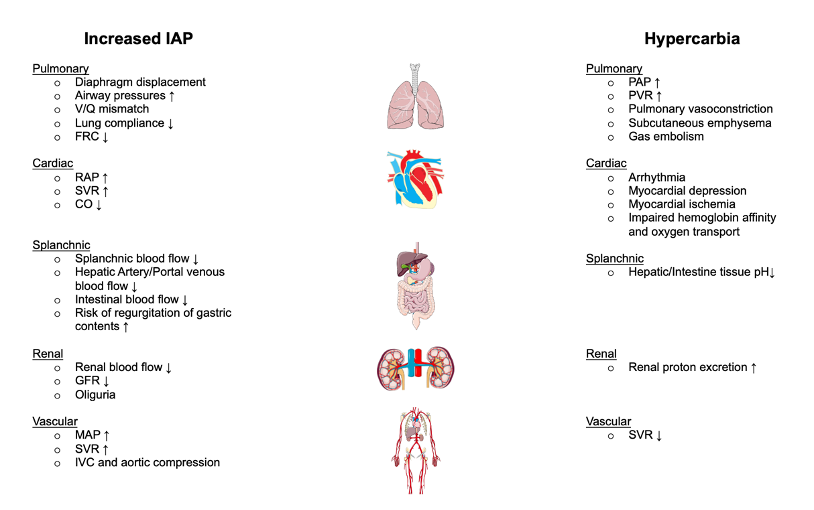
Figure 2. Detrimental effects of increased intraabdominal pressure and hypercarbia during laparoscopic surgeries. Adapted from Atkinson T, et al. Cardiovascular and ventilatory consequences of laparoscopic surgery. Circulation: 2017; 135:700-10.1
Anesthetic Considerations
Type of Anesthesia3,5,6
- General anesthesia is the standard of care to maintain ventilatory support. However, there have been reports of laparoscopic and robotic surgery being performed in patients receiving neuraxial anesthesia with a sensory level up to T4-T6.
Airway Management2,4
- An endotracheal tube is preferred over a supraglottic airway. This allows for increased ventilatory pressures and the addition of positive end-expiratory pressure (PEEP), which are often needed.
Monitoring2,4
- Standard American Society of Anesthesiologists monitors should be used.
- Arms are usually tucked. If frequent lab draws are necessary, or if there is concern about hemodynamic status, an arterial line placement can also be considered.
Maintenance of Anesthesia4,8,10
- Volatile anesthetics or intravenous anesthetic agents may be used for the maintenance of general anesthesia. The use of nitrous oxide is controversial; however, given the risk of postoperative nausea and vomiting, and bowel distention, nitrous oxide is best avoided.
- Neuromuscular blockade is utilized during induction. Continuation during the surgery is controversial. Potential benefits of redosing include improved surgical exposure from diaphragmatic movement and prevention of patient injury while the robot is docked.
Mechanical Ventilation2,8,9
- A lung-protective strategy of 6-8 mL/kg of ideal body weight and positive end-expiratory pressure (PEEP) 5-10 cm H2O is often utilized. Titration can be based on driving pressure and respiratory compliance.
- During laparoscopic robotic surgery, the driving pressures are distributed more to the chest wall and less to the lungs. Therefore, it may be necessary to accept higher peak airway and driving pressures to prevent lung collapse and maintain adequate ventilation.
- The end-tidal CO2 goal should be ~40 mmHg. Permissive hypercapnia is acceptable to avoid barotrauma. If extreme hypercarbia or uncontrolled acidosis occurs, insufflation pressure can be interrupted to reduce CO2.
- Oxygen saturation should be maintained above 90%.
Fluid Management4,6,8,9
- A balanced crystalloid solution should be utilized, and goal-directed fluid administration should be maintained. Excessive fluid administration can lead to facial, pharyngeal, and laryngeal edema, which is made worse in Trendelenburg positioning.
- Surrogate markers, including heart rate, blood pressure, central venous pressure, and urine output, are poor predictors of intravascular fluid status, especially in pneumoperitoneum.
- Arterial waveform monitoring or utilization of a FloTrac system can be considered in select patients to evaluate pulse pressure or stroke volume variation, stroke volume, or cardiac output.
Pain Management4,5,11
- Pain results from both somatic (port sites) and visceral (peritoneal stretch and manipulation of abdominal tissues) stimuli.
- A multimodal analgesic regimen, including acetaminophen and nonsteroidal anti-inflammatory drugs, is often recommended to limit opioid use.
- Local anesthetics can be injected into the port sites.
- For laparoscopic-assisted or hybrid approaches, regional anesthesia (transversus abdominis plane blocks) can be offered
- Intraperitoneal local anesthetic deposition has not been routinely recommended.
- Neuraxial anesthesia is often unnecessary.
Postoperative Nausea and Vomiting (PONV) Prophylaxis4
- Laparoscopy is an independent risk factor for PONV. Therefore, prophylactic antiemetic administration is recommended.
Positioning4,6
- Face protection from robot arms should be maintained.
- Foam pads or a protective metal barrier over the face are often used. The anesthesia provider must also be vigilant in recognizing pressure injuries on the head and face and accidental extubation by moving robot arms.
- Potential patient positioning options include extreme Trendelenburg or reverse Trendelenburg, lateral decubitus, lithotomy, or flexed position.
- If the robot is docked, the operating room (OR) table must not move to avoid injury from the robot arms.
- Robotic surgeries are often longer in duration than open procedures, so special attention must be paid to padding pressure points.
- Positioning devices that are often used include nonslip pads, cross-body taping, and shoulder supports.
- There is a higher risk of iatrogenic injury with robots because tactile feedback that surgeons receive from open or laparoscopically performed procedures is no longer present.
- To facilitate surgical exposure, the placement of an orogastric tube for stomach decompression should be considered.
Intraoperative Complications1,3,4
- Complications are mostly related to cardiopulmonary physiologic effects, surgical instrumentation, patient positioning, or due to carbon dioxide insufflation.
- Hemodynamic effects associated with insufflation can include increased abdominal pressure resulting in IVC compression. Additionally, upon the initiation of insufflation, there can be a pronounced vagal response leading to cardiac arrest.
- Acidosis from the hypercarbia itself can cause a decrease in cardiac contractility or a decrease in systemic vascular resistance.
- Other hemodynamic concerns can also be related to hemorrhage, positional blood pooling, gas embolism, or capnomediastinum/capnopericardium, which can result in tamponade physiology.
- Pulmonary complications are mainly due to impaired respiratory mechanics during insufflation and/or surgical injury to the diaphragm or lung.
- Complications related to changes in patient position include endobronchial intubation, endotracheal tube obstruction, or atelectasis.
- During Veress needle placement or port access, complications include solid organ injury or hemorrhage, which may not be as obvious due to limitations of visualization of the surgical field.
- Additional complications of insufflation include subcutaneous emphysema if a Veress needle or trocar is improperly placed during extraperitoneal or upper abdominal laparoscopy. Signs include crepitus over the abdomen, chest, clavicles, and neck. Risk factors for the development of subcutaneous emphysema include prolonged surgery lasting more than 200 minutes, the use of six or more ports, age greater than 65 years, and Nissen fundoplication surgery.
- Capnothorax can result from a peritoneal breach of the Veress needle or peritoneal ports, retroperitoneal insufflation, dissection around the diaphragm, the passage of gas through the pleuroperitoneal hiatus, or congenital defects of the diaphragm. Signs include increased airway pressure, hypoxemia, hypercapnia, inequality of chest expansion, bulging diaphragm, and emphysema of the head and neck.
- Subcutaneous emphysema or capnothorax often resolves with discontinuation of insufflation. However, attention must be paid to potential airway compromise or significant hemodynamic changes. Treatment ranges from conservative therapy, including no treatment, to decompression of the capnothorax via chest tube placement or open surgery. Regardless, if there is significant subcutaneous emphysema or capnothorax, patients should be closely monitored for eventual resolution of symptoms.
- Positioning complications related to steep Trendelenburg positioning include conjunctival, nasal, and laryngopharyngeal edema, postoperative visual loss from ischemic optic neuropathy, position-related nerve injury, or compartment syndrome.
References
- Atkinson T, Giraud G, Togioka B, et al. Cardiovascular and ventilatory consequences of laparoscopic surgery. Circulation: 2017; 135:700-10. PubMed
- Ellis DB, Albrecht M. Anesthesia for robotic surgery. In: Gropper M, et al (eds). Miller’s Anesthesia. 9th edition. Philadelphia, PA; Elsevier; 2020: 2236-2250.e3.
- Gokdemir BN, Cekmen N. Laparoscopic surgery and anesthesia. JARSS. 2023;31(3):173-87. Link
- Hayden P, Cowman S. Anaesthesia for laparoscopic surgery. Continuing Education in Anaesthesia Critical Care & Pain. 2011;11(5):177-180. Link
- Bajwa SJS Kulshrestha A. Anaesthesia for laparoscopic surgery: General vs regional anaesthesia. J Minim Access Surg. 2016. 12(1): 4-9. Link
- Joshi GP, Cunningham AJ. Anesthesia for laparoscopic and robotic surgery. In: Barash PG, Cullen BF, Steolting RK, et al. Clinical Anesthesia. 7th edition. Philadelphia, PA; Lippincott, Williams & Wilkins; 2013: 1257.
- Meininger D, Westphal K, Bremerich DH, et al. Effects of posture and prolonged pneumoperitoneum on hemodynamic parameters during laparoscopy. World J Surg. 2008; 32:1400. PubMed
- Meininger D, Zwissler B, Byhahn C, et al. Impact of overweight and pneumoperitoneum on hemodynamics and oxygenation during prolonged laparoscopic surgery. World J Surg. 2006; 30:520. PubMed
- O'Malley C, Cunningham AJ. Physiologic changes during laparoscopy. Anesthesiol Clin North Am. 2001; 19:1. PubMed
- Martini CH, Boon M, Bevers RF, et al. Evaluation of surgical conditions during laparoscopic surgery in patients with moderate vs deep neuromuscular block. Br J Anaesth. 2014; 112:498. PubMed
- Joshi GP, Kehlet H. Postoperative pain management in the era of ERAS: An overview. Best Pract Res Clin Anaesthesiol. 2019; 33:259. PubMed
Copyright Information
This work is licensed under a Creative Commons Attribution-NonCommercial-NoDerivatives 4.0 International License.