Copy link
Pediatric Ventilation: Anesthesia Ventilators
Last updated: 08/23/2023
Key Points
- Technologies used in modern anesthesia ventilators include bellows, piston, turbine, and volume manifold designs. These modern anesthesia ventilator designs are generally reliable and effective for pediatric ventilation.
- Since compliance of the breathing circuit can alter both delivered and measured tidal volume, compliance compensation is essential to achieve accurate and precise volume delivery and measurement.
- Careful attention to performing the pre-use test properly prior to each procedure is required to achieve effective intraoperative ventilation.
- Bedside monitoring of oxygenation, ventilation, and lung compliance is essential for optimizing ventilation for the individual patient, irrespective of the underlying technology.
Types of Anesthesia Ventilators
- The goal of delivering precisely controlled tidal volumes can be achieved using various types of anesthesia ventilators. Anesthesia ventilators differ from intensive care unit ventilators in that the patient gas is separated from the method of developing inspiratory pressure.
- Piston (Figure 1A) and turbine (Figure 1B) ventilators utilize electrical power to provide the inspiratory flow. Bellows (Figure 1C) and volume reflector (Figure 1D) ventilators are pneumatically driven, using high-pressure gas from the wall supply to drive gas towards the patient’s lungs. While the rise and fall of the bellows is traditionally used as an indicator of ventilator function1, the presence of leak and disconnect alarms on most modern anesthesia workstations based upon multiple sensors, including pressure, flow, and CO2 makes this visual confirmation less relevant.
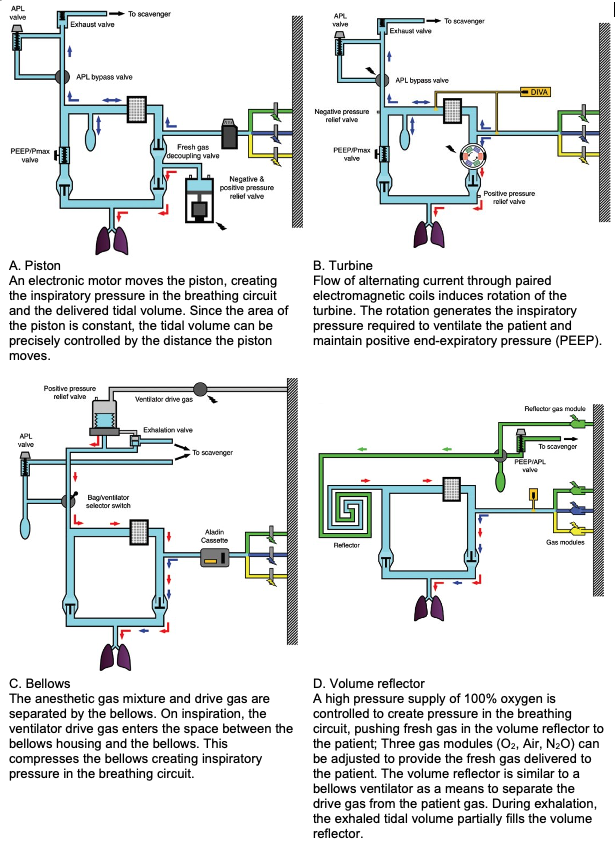
Figure 1. Types of anesthesia ventilators and their configurations in the anesthesia circuit. Wall gas supply is depicted by colored lightning bolts (O2 green, N2O blue, air yellow). Blue arrows represent fresh gas flow, and red arrows the flow generated by the ventilator. Inspiratory valves are open and expiratory valves are closed as the systems are in the inspiratory phase of a mechanical breath. Adapted with permission from Hendrickx JFA, De Wolf AM. The anesthesia workstation. Quo Vadis? Anesth Analg. 2018;127(3):671-5.
Compliance Compensation
- Compliance compensation is important for i) ensuring that the set tidal volume is delivered to the patient’s airway, and ii) ensuring that the exhaled tidal volume measurement estimates the volume delivered to the airway as accurately as possible.
- The compliance of a breathing system is the change in volume per unit change in pressure measured in mL/cm H2O. When applied to the breathing circuit, compliance results from a combination of gas compression in the circuit and the distensibility of the tubing.
- As the ventilator applies the inspiratory pressure, the breathing system expands and the gas within the system is compressed such that a portion of the delivered tidal volume is lost to overcome the circuit compliance. This can cause a discrepancy between the set tidal volume and the actual tidal volume delivered to the patient unless compliance compensation is a feature of the ventilator and working properly. This discrepancy becomes more significant in smaller patients since it represents a greater fraction of the intended tidal volume than in larger patients. The smaller the patient, the more likely it is that delivered volume discrepancies due to circuit compliance will lead to hypoventilation.2
- Modern anesthesia machines compensate for circuit compliance by:
- measuring circuit compliance during the pre-use checkout;
- adjusting tidal volumes delivered by the ventilator to account for the volume loss due to circuit compliance; and
- precisely measuring both inspiratory and expiratory tidal volumes.3
- The pre-use compliance test is essential to ensure the proper function of compliance compensation. During this test, the ventilator applies a set pressure to the breathing circuit and measures the volume of gas required to achieve that pressure. This measurement is used to determine the additional tidal volume needed to compensate for circuit compliance.
- Compliance compensation is only effective if the pre-use test is performed properly:
- The test should be performed prior to each case.
- All components added to the breathing circuit (such as humidifiers) to be used for the anesthetic must be in the circuit – adding and removing components after the test will compromise the accuracy of compensation.
- The circuit should be in the final configuration required for the case – expanding or collapsing an adjustable length circuit will alter the circuit compliance and impact the accuracy of tidal volume measurement and delivery.
Fresh Gas Flow and Tidal Volume
- Fresh gas flows continuously into the breathing system with the potential to alter tidal volume during inspiration. This interaction between fresh gas flow and tidal volume can make it difficult to manage ventilation precisely, especially in pediatric patients.
- In workstations equipped with fresh gas decoupling, closure of the decoupling valve during the inspiratory phase diverts fresh gas into the reservoir bag, ensuring that tidal volume is independent of fresh gas flow (Figure 1A).
- Fresh gas compensation is another engineering solution to this problem. An integrated flow sensor detects the amount of fresh gas flow and adjusts the delivered tidal volume accordingly.
Monitoring to Optimize Ventilation
- The potential for ventilator-induced lung injury exists even during short periods of perioperative ventilation.4 This injury can occur from atelectotrauma, volutrauma, barotrauma, and hyperoxia.5 The risk, however, can be reduced by thoughtful intraoperative ventilator strategies.
- The goals of optimal ventilation are:
- optimal arterial oxygen tension (PaO2) at the least possible fraction of inspired oxygen concentration (FiO2);
- acceptable arterial carbon dioxide tension (PaCO2); and
- adequate tidal volumes with the lowest possible inspiratory pressures.
- Information from various monitors can be used to achieve these goals.
- Arterial blood gas sampling is the gold standard for measuring PaO2 and PaCO2, but it is not a continuous monitor and requires placement of an arterial catheter.
- Pulse oximetry and capnography are good surrogates for arterial blood gas measurement but have some limitations. Pulse oximetry is most meaningful when the breathing gas contains the lowest inspired oxygen concentration that is clinically feasible. If the FiO2 is excessively high, oxyhemoglobin saturation will remain high even when oxygen exchange is impaired. There is always a gradient between the end-tidal and arterial CO2. This gradient can be large in small patients especially if the dead-space to tidal volume ratio is not managed by careful attention to apparatus deadspace.
- Finally, monitoring lung compliance and treating causes of adverse compliance, such as atelectasis, can help to reduce inspiratory pressures. By setting a baseline pressure-volume loop and comparing each breath against that loop, the clinician can detect early decreases in lung compliance and reverse them with maneuvers such as recruitment or applying positive end-expiratory pressure.
References
- Hendrickx JFA, De Wolf AM. The anesthesia workstation. Quo Vadis? Anesth Analg. 2018;127(3):671-5. PubMed
- Feldman JM. Optimal ventilation of the anesthetized pediatric patient. Anesth Analg. 2015;120(1):165-75. PubMed
- Spaeth J, Schumann S, Humphreys S. Understanding pediatric ventilation in the operative setting. Part I: Physical principles of monitoring in the modern anesthesia workstation. Paediatr Anaesth. 2022;32(2):237-46. PubMed
- Ball L, Almondo C, Pelosi P. Perioperative lung protection: General mechanisms and protective approaches. Anesth Analg. 2020;131(6):1789-98. PubMed
- Heath C, Hauser N. Is there a role for lung‐protective ventilation in healthy children? Paediatr Anaesth. 2021;32(2):278-85. PubMed
Copyright Information
This work is licensed under a Creative Commons Attribution-NonCommercial-NoDerivatives 4.0 International License.